Description
Polymer Composites for Electrical Engineering
IEEE Press Series
Coordinators: Huang Xingyi, Tanaka Toshikatsu
Language: English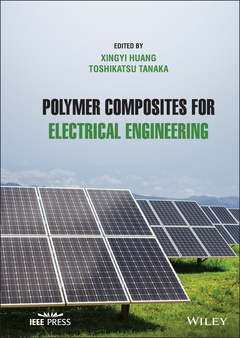
Subject for Polymer Composites for Electrical Engineering:
Support: Print on demand
Description
/li>Contents
/li>Biography
/li>
Explore the diverse electrical engineering application of polymer composite materials with this in-depth collection edited by leaders in the field
Polymer Composites for Electrical Engineering delivers a comprehensive exploration of the fundamental principles, state-of-the-art research, and future challenges of polymer composites. Written from the perspective of electrical engineering applications, like electrical and thermal energy storage, high temperature applications, fire retardance, power cables, electric stress control, and others, the book covers all major application branches of these widely used materials.
Rather than focus on polymer composite materials themselves, the distinguished editors have chosen to collect contributions from industry leaders in the area of real and practical electrical engineering applications of polymer composites. The book?s relevance will only increase as advanced polymer composites receive more attention and interest in the area of advanced electronic devices and electric power equipment.
Unique amongst its peers, Polymer Composites for Electrical Engineering offers readers a collection of practical and insightful materials that will be of great interest to both academic and industrial audiences. Those resources include:
Perfect for postgraduate students and researchers working in the fields of electrical, electronic, and polymer engineering, Polymer Composites for Electrical Engineering will also earn a place in the libraries of those working in the areas of composite materials, energy science and technology, and nanotechnology.
List of Contributors xv
Preface xix
1 Polymer Composites for Electrical Energy Storage 1
Yao Zhou
1.1 Introduction 1
1.2 General Considerations 1
1.3 Effect of Nanofiller Dimension 3
1.4 Orientation of Nanofillers 7
1.5 Surface Modification of Nanofillers 11
1.6 Polymer Composites with Multiple Nanofillers 13
1.7 Multilayer-structured Polymer Composites 16
1.8 Conclusion 19
References 21
2 Polymer Composites for Thermal Energy Storage 29
Jie Yang, Chang-Ping Feng, Lu Bai, Rui-Ying Bao, Ming-Bo Yang, and Wei Yang
2.1 Introduction 29
2.2 Shape-stabilized Polymeric Phase Change Composites 32
2.2.1 Micro/Nanoencapsulated Method 33
2.2.2 Physical Blending 35
2.2.3 Porous Supporting Scaffolds 36
2.2.4 Solid–Solid Composite PCMs 37
2.3 Thermally Conductive Polymeric Phase Change Composites 39
2.3.1 Metals 40
2.3.2 Carbon Materials 41
2.3.3 Ceramics 41
2.4 Energy Conversion and Storage Based on Polymeric Phase Change Composites 42
2.4.1 Electro-to-Heat Conversion 42
2.4.2 Light-to-Heat Conversion 45
2.4.3 Magnetism-to-Heat Conversion 47
2.4.4 Heat-to-Electricity Conversion 48
2.5 Emerging Applications of Polymeric Phase Change Composites 48
2.5.1 Thermal Management of Electronics 49
2.5.2 Smart Textiles 50
2.5.3 Shape Memory Devices 51
2.6 Conclusions and Outlook 51
Acknowledgments 52
References 52
3 Polymer Composites for High-Temperature Applications 63
Sen Niu, Lixue Zhu, Qiannan Cai, and Yunhe Zhang
3.1 Application of Polymer Composite Materials in High-Temperature Electrical Insulation 63
3.1.1 High-Temperature-Resistant Electrical Insulating Resin Matrix 63
3.1.1.1 Silicone Resins 64
3.1.1.2 Polyimide 64
3.1.1.3 Polyether Ether Ketone 65
3.1.1.4 Polybenzimidazole 65
3.1.1.5 Polyphenylquinoxaline 65
3.1.1.6 Benzoxazine 66
3.1.2 Modification of Resin Matrix with Reinforcements 66
3.1.2.1 Mica 66
3.1.2.2 Glass Fiber 66
3.1.2.3 Inorganic Nanoparticles 67
3.1.3 Modifications in the Thermal Conductivity of Resin Matrix 67
3.1.3.1 Mechanism of Thermal Conductivity 68
3.1.3.2 Intrinsic High Thermal Conductivity Insulating Material 68
3.1.3.3 Filled High Thermal Conductivity Insulating Material 69
3.2 High-Temperature Applications for Electrical Energy Storage 70
3.2.1 General Considerations for High-Temperature Dielectrics 70
3.2.2 High-Temperature-Resistant Polymer Matrix 71
3.2.3 Polymer Composites for High-Temperature Energy Storage Applications 71
3.2.4 Surface Modification of Nanocomposite for High-Temperature Applications 72
3.2.5 Sandwich Structure of Nanoparticles for High-Temperature Applications 75
3.3 Application of High-Temperature Polymer in Electronic Packaging 77
3.3.1 Synthesis of Low Dielectric Constant Polymer Materials Through Molecular Structure Design 80
3.3.1.1 Fluorine-Containing Low Dielectric Constant Polymer 80
3.3.1.2 Low Dielectric Constant Polymer Material Containing Nonpolar Rigid Bulk Group 81
3.3.2 High-Temperature-Resistant Low Dielectric Constant Polymer Composite Material 82
3.3.2.1 Low Dielectric Constant Polyoxometalates/Polymer Composite 83
3.3.2.2 Low Dielectric Constant POSS/Polymer Composite 85
3.4 Application of Polymer Composite Materials in the Field of High-Temperature Wave-Transmitting and Wave-Absorbing Electrical Fields 86
3.4.1 Wave-Transmitting Materials 88
3.4.1.1 The High-Temperature Resin Matrix 88
3.4.1.2 Reinforced Materials 89
3.4.2 Absorbing Material 89
3.4.2.1 The High-Temperature Resin Matrix 90
3.4.2.2 Inorganic Filler 90
3.5 Summary 91
References 92
4 Fire-Retardant Polymer Composites for Electrical Engineering 99
Zhi Li, En Tang, and Xue-Meng Cao
4.1 Introduction 99
4.2 Fire-Retardant Cables and Wires 100
4.2.1 Fundamental Overview 100
4.2.2 Understanding of Fire-Retardant Cables and Wires 101
4.2.2.1 Polyethylene Composites 101
4.2.2.2 Ethylene-Vinyl Acetate (EVA) Copolymer 103
4.2.2.3 Polyvinyl Chloride Composites 105
4.2.2.4 Other Polymers 108
4.3 Fire-Retardant Polymer Composites for Electrical Equipment 109
4.3.1 Fundamental Overview 109
4.3.2 Understanding of Fire-Retardant Polymer Composites for Electrical Equipment 110
4.3.2.1 HIPS and ABS Composites 110
4.3.2.2 PC/ABS Composites 112
4.3.2.3 PC Composites 115
4.3.2.4 PBT Composites 116
4.4 Fire-Retardant Fiber Reinforced Polymer Composites 117
4.4.1 Fundamental Overview 117
4.4.2 Understanding of Fire-Retardant Fiber Reinforced Polymer Composites 118
4.4.2.1 Reinforced PBT and PET Composites 118
4.5 Conclusion and Outlook 118
References 119
5 Polymer Composites for Power Cable Insulation 123
Yoitsu Sekiguchi
5.1 Introduction 123
5.2 Trend in Nanocomposite Materials for Cable Insulation 125
5.2.1 Overview 125
5.2.2 Polymer Materials as Matrix Resin 125
5.2.3 Fillers 128
5.2.4 Nanocomposites 130
5.2.4.1 XLPE Nanocomposites 131
5.2.4.2 PP Nanocomposites 131
5.2.4.3 Nanocomposite with Cluster/Cage Molecule 131
5.2.4.4 Copolymer and Polymer Blend 131
5.3 Factors Influencing Properties 138
5.4 Issues in Nanocomposite Insulation Materials Research 139
5.5 Understanding Dielectric and Insulation Phenomena 140
5.5.1 Electromagnetic Understanding 140
5.5.2 Understanding Space Charge Behavior by Q(t) Method 141
References 146
6 Semi-conductive Polymer Composites for Power Cables 153
Zhonglei Li, Boxue Du, Yutong Zhao, and Tao Han
6.1 Introduction 153
6.1.1 Function of Semi-conductive Composites 153
6.1.2 Development of Semi-conductive Composites 154
6.2 Conductive Mechanism of Semi-conductive Polymer Composites 155
6.2.1 Percolation Theory 157
6.2.2 Tunneling Conduction Theory 157
6.2.3 Mechanism of Positive Temperature Coefficient 158
6.3 Effect of Polymer Matrix on Semi-conductivity 159
6.3.1 Thermoset Polymer Matrix 159
6.3.2 Thermoplastic Polymer Matrix 162
6.3.3 Blended Polymer Matrix 163
6.4 Effect of Conductive Fillers on Semi-conductivity 165
6.4.1 Carbon Black 165
6.4.2 Carbonaceous Fillers with One- and Two-Dimensions 166
6.4.3 Secondary Filler for Carbon Black Filled Composites 167
6.5 Effect of Semi-conductive Composites on Space Charge Injection 169
6.6 Conclusions 172
References 173
7 Polymer Composites for Electric Stress Control 179
Muneaki Kurimoto
7.1 Introduction 179
7.2 Functionally Graded Solid Insulators and Their Effect on Reducing Electric Field Stress 179
7.3 Practical Application of ε-FGMs to GIS Spacer 181
7.4 Application to Power Apparatus 182
References 188
8 Composite Materials Used in Outdoor Insulation 191
Wang Xilin, Jia Zhidong, and Wang Liming
8.1 Introduction 191
8.2 Overview of SIR Materials 192
8.2.1 RTV Coatings 193
8.2.2 Composite Insulators 195
8.2.3 Liquid Silicone Rubber (LSR) 196
8.2.4 Aging Mechanism and Condition Assessment of SIR Materials 197
8.3 New External Insulation Materials 198
8.3.1 Anti-icing Semiconductor Materials 199
8.3.2 Hydrophobic CEP 201
8.4 Summary 202
References 203
9 Polymer Composites for Embedded Capacitors 207
Shuhui Yu, Suibin Luo, Riming Wang, and Rong Sun
9.1 Introduction 207
9.1.1 Development of Embedded Technology 207
9.1.2 Dielectric Materials for Commercial Embedded Capacitors 210
9.2 Researches on the Polymer-Based Dielectric Nanocomposites 213
9.2.1 Filler Particles 213
9.2.2 Epoxy Matrix 216
9.2.2.1 Modification to Improve Dielectric Properties 219
9.2.2.2 Modification to Improve Mechanical Properties 221
9.3 Fabrication Process of Embedded Capacitors 224
9.4 Reliability Tested of Embedded Capacitor Materials 229
9.5 Conclusions and Perspectives 230
References 230
10 Polymer Composites for Generators and Motors 235
Hirotaka Muto, Takahiro Umemoto, and Takahiro Mabuchi
10.1 Introduction 235
10.2 Polymer Composite in High-Voltage Rotating Machines 236
10.3 Ground Wall Insulation 237
10.3.1 Mica/Epoxy Insulation 237
10.3.2 Electrical Defect in the Insulation of Rotating Machines and Degradation Mechanism 238
10.3.3 Insulation Design and V-t Curve 239
10.4 Polymer Nanocomposite for Rotating Machine 240
10.4.1 Partial Discharge Resistance and a Treeing Lifetime of Nanocomposite as Material Property 241
10.4.1.1 PD Resistance 241
10.4.1.2 Electrical Treeing Lifetime 242
10.4.2 Breakdown Lifetime Properties of Realistic Insulation Defect in Rotating Machine 244
10.4.2.1 Voltage Endurance Test of Void Defect 245
10.4.2.2 Voltage Endurance Test in Mica/Epoxy Nanocomposite-Layered Structure 247
10.4.2.3 V-t Curves in Coil Bar Model with Mica/Epoxy Nanocomposite Insulation 248
10.5 Stress-Grading System of Rotating Machines 252
10.5.1 Silicon Carbide Particle-Loaded Nonlinear-Resistive Materials 252
10.5.2 End-turn Stress-Grading System of High-Voltage Rotating Machines 253
References 255
11 Polymer Composite Conductors and Lightning Damage 259
Xueling Yao
11.1 Lightning Environment and Lightning Damage Threat to Composite-Based Aircraft 259
11.1.1 The Lightning Environment 259
11.1.1.1 Formation of Lightning 259
11.1.2 Lightning Test Environment of Aircrafts 261
11.1.2.1 Zone 1 262
11.1.2.2 Zone 2 263
11.1.2.3 Zone 3 263
11.1.2.4 Current Component A – First Return Strike 264
11.1.2.5 Current Component Ah – Transition Zone First Return Strike 264
11.1.2.6 Current Component B – Intermediate Current 264
11.1.2.7 Current Component C – Continuing Current 264
11.1.2.8 Component C* – Modified Component C 264
11.1.2.9 Current Component D – Subsequent Strike Current 266
11.1.3 Waveform Combination in Different Lightning Zones for Lightning Direct Effect Testing 269
11.1.4 Application of CFRP Composites in Aircraft 269
11.2 The Dynamic Conductive Characteristics of CFRP 271
11.2.1 A Review of the Research on the Conductivity of CFRP 271
11.2.2 The Testing Methods 272
11.2.2.1 Specimens 272
11.2.2.2 The Test Fixture 273
11.2.2.3 Lightning Impulse Generator and Lightning Waveforms 274
11.2.3 The Experimental Results of the Dynamic Impedance of CFRP 275
11.2.3.1 The Nondestructive Lightning Current Test 275
11.2.3.2 The Applied Lightning Current Impulse and the Response Voltage Impulse 278
11.2.3.3 Equivalent Conductivity of CFRP Laminates Under Different Lightning Impulses 280
11.2.3.4 Equivalent Conductivity of CFRP Laminates with Different Laminated Structures 282
11.2.4 The Discussion of the Dynamic Conductive Characteristics of CFRP 282
11.2.4.1 The Conduction Path of the CFRP Laminate Under a Lightning Current Impulse 282
11.2.4.2 Dynamic Conductance of CFRP Laminate 284
11.2.4.3 The Inductive Properties of CFRP Laminates 286
11.2.4.4 Equivalent Conductivity of CFRP Laminates Subjected to Lightning Current Impulses with Higher Intensity 288
11.3 The Lightning Strike-Induced Damage of CFRP Strike 289
11.3.1 Introduction of the Lightning Damage of CFRP 289
11.3.2 Single Lightning Strike-Induced Damage 290
11.3.2.1 Experimental Setup for Single Lightning Strike Test 290
11.3.2.2 Experimental Results of Single Lightning Strike-Induced Damage 292
11.3.2.3 Evaluation for Single Lightning Strike-Induced Damage 297
11.3.3 Multiple Lightning Strikes-Induced Damage 300
11.3.3.1 Experimental Method for Multiple Consecutive Lightning Strike Tests 300
11.3.3.2 Experimental Results of Multiple Lightning Damage 303
11.3.3.3 Multiple Lightning Damage Areas and Depths of CFRP Laminates 308
11.3.3.4 Analysis for Multiple Lightning Damage of CFRP Laminates 309
11.3.3.5 Evaluation for Multiple Lightning Damage of CFRP Laminates 313
11.4 The Simulation of Lightning Strike-Induced Damage of CFRP 319
11.4.1 Overview of Lightning Damage Simulation Researches 319
11.4.2 Establishment of the Coupled Thermal-Electrical Model 321
11.4.2.1 Finite Element Model 321
11.4.2.2 Simulated Lightning Component A 322
11.4.2.3 Pyrolysis Degree Calculation 322
11.4.2.4 Dynamic Conductive Properties 322
11.4.2.5 Pyrolysis-Dependent Material Parameters 323
11.4.3 Simulation Physical Fields of Lightning Current on CFRP Laminates 323
11.4.3.1 Temperature and Pyrolysis Fields 323
11.4.3.2 Mechanical Analysis 325
11.4.4 Simulated Lightning Damage Results 325
11.4.4.1 Numerical Criterion for Lightning Damage 325
11.4.4.2 In-Plane Lightning Damage Evaluation 327
11.4.4.3 In-Depth Lightning Damage Evaluation 331
References 331
12 Polymer Composites for Switchgears 339
Takahiro Imai
12.1 Introduction 339
12.2 History of Switchgear 340
12.3 Typical Insulators in Switchgears 342
12.3.1 Epoxy-based Composite Insulators 342
12.3.2 Insulator-Manufacturing Process 343
12.3.2.1 Vacuum Casting Method 344
12.3.2.2 Automatic Pressure Gelation Method 344
12.3.2.3 Vacuum Pressure Impregnation Method 345
12.4 Materials for Epoxy-based Composites 345
12.4.1 Epoxy Resins 345
12.4.2 Hardeners 346
12.4.3 Inorganic Fillers and Fibers 347
12.4.4 Silane Coupling Agents 348
12.4.5 Fabrication of Epoxy-based Composites 349
12.5 Properties of Epoxy-based Composites 351
12.5.1 Necessary Properties of Epoxy-based Composites for Switchgears 351
12.5.2 Resistance to Thermal Stresses 352
12.5.2.1 Glass Transition Temperature 352
12.5.2.2 Coefficient of Thermal Expansion (CTE) 354
12.5.3 Resistances to Electrical Stresses 356
12.5.3.1 Short-term Insulation Breakdown 356
12.5.3.2 Long-term Insulation Breakdown (V-t Characteristics) 357
12.5.3.3 Relative Permittivity and Resistivity 359
12.5.4 Resistances to Ambient Stresses 360
12.5.4.1 Resistance to SF6 Decomposition Gas 360
12.5.4.2 Water Absorption 361
12.5.5 Resistances to Mechanical Stresses 362
12.5.5.1 Flexural and Tensile Strength 362
12.5.5.2 Creep 363
12.5.6 International Standards for Evaluation of Composites 363
12.6 Advances of Epoxy-based Composites for Switchgear 365
12.6.1 Nanocomposites 365
12.6.2 High Thermal Conductive Composites 366
12.6.3 Biomass Material-Based Composites 367
12.6.4 Functionally Graded Materials 368
12.6.5 Estimate of Remaining Life of Composites 370
12.7 Conclusion 372
References 373
13 Glass Fiber-Reinforced Polymer Composites for Power Equipment 377
Yu Chen
13.1 Overview 377
13.2 Glass Fiber-Reinforced Polymer Composites 378
13.2.1 Fibers 378
13.2.1.1 Chemical Description 378
13.2.1.2 Classification of Glass Fibers 380
13.2.1.3 Properties of Glass Fiber 380
13.2.1.4 Glass Fabrics 380
13.2.1.5 Advantages and Disadvantages 381
13.2.1.6 Common Manufacturing Methods 383
13.2.1.7 Applications of Glass Fiber in Various Industries 384
13.2.2 Polymers 386
13.2.2.1 Epoxy 386
13.2.2.2 Polyester (Thermosetting) 386
13.2.2.3 Phenolic 387
13.2.3 Manufacturing Methods 388
13.2.4 Specifications of Several Kinds of GFRP Materials 393
13.2.4.1 Rigid Laminated Sheets 393
13.2.4.2 Industrial Rigid Round Laminated Rolled Tubes 394
13.2.4.3 Insulated Pipe 394
13.2.4.4 Insulated Pull Rod 394
13.3 Application of Glass Fiber-Reinforced Polymer Composites 396
13.3.1 Laminated Sheets 396
13.3.2 Composite Long Rod Insulators 398
13.3.3 UHV-Insulated Pull Rod for GIS 400
13.3.4 Composite Pole 403
13.3.5 Aluminum Conductor Composite Core in an Overhead Conductor 404
13.3.6 Composite Station Post Insulators 405
13.3.7 Composite Hollow Insulators 407
13.3.8 Composite Crossarms 407
Bibliography 414
Index 419
Xingyi Huang, PhD, is Professor and Deputy Director of the Shanghai Key Laboratory of Electrical Insulation and Thermal Aging at the Shanghai Jiao Tong University in China. He is an Associate Editor of IEEE Transactions on Dielectric and Electrical Insulation, as well as an Associate Editor of IEEE High Voltage.
Toshikatsu Tanaka, PhD, is Chairman of the IEEJ Committee on New Dielectric Materials. He is Vice President of the Central Research Institute of the Electric Power Industry and is a recipient of the Japanese Ministry of Science and Technology Prize.