Description
Performance Evaluation and Design of Flight Vehicle Control Systems
Author: Falangas Eric T.
Language: English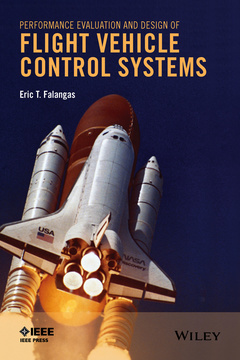
Subject for Performance Evaluation and Design of Flight Vehicle...:
Keywords
flight vehicle modelling; static performance analysis; static stability; control authority; tail-wags-dog; maneuverability; structural flexibility; flex modes; propellant sloshing; flight control design; LQR; H-infinity; trimming; mixing-logic; control effectors combination; contour plots; vector diagrams; angle of attack estimator; lateral control departure parameter; LCDP; Cn-beta-dynamic; TVC; engine throttling; aero-surfaces; RCS; actuators; time to double amplitude; hinge moments; spin departure; spin susceptibility; Eric Falangas
432 p. · 15.8x23.6 cm · Hardback
Description
/li>Contents
/li>Biography
/li>
- Graphical methods for analysing vehicle performance
- Methods for trimming deflections of a vehicle that has multiple types of effectors
- Presents a parameters used for speedily evaluating the performance, stability, and controllability of a new flight vehicle concept along a trajectory or with fixed flight conditions
Acknowledgments xiii
Introduction 1
1 Description of the Dynamic Models 7
1.1 Aerodynamic Models, 8
1.2 Structural Flexibility, 9
1.3 Propellant Sloshing, 10
1.4 Dynamic Coupling between Vehicle, Actuators, and Control Effectors, 12
1.5 Control Issues, 13
1.6 Coordinate Axes, 15
Nomenclature, 16
2 Nonlinear Rigid-Body Equations Used in 6-DOF Simulations 19
2.1 Force and Acceleration Equations, 19
2.2 Moment and Angular Acceleration Equations, 21
2.3 Gravitational Forces, 22
2.4 Engine TVC Forces, 22
2.5 Aerodynamic Forces and Moments, 24
2.6 Propellant Sloshing Using the Pendulum Model, 28
2.7 Euler Angles, 29
2.8 Vehicle Altitude and Cross-Range Velocity Calculation, 30
2.9 Rates with Respect to the Stability Axes, 30
2.10 Turn Coordination, 31
2.11 Acceleration Sensed by an Accelerometer, 31
2.12 Vehicle Controlled with a System of Momentum Exchange Devices, 32
2.13 Spacecraft Controlled with Reaction Wheels Array, 33
2.14 Spacecraft Controlled with an Array of Single-Gimbal CMGs, 37
2.14.1 Math Model of a SGCMG Array, 38
2.14.2 Steering Logic for a Spacecraft with SGCMGs, 42
3 Linear Perturbation Equations Used in Control Analysis 47
3.1 Force and Acceleration Equations, 47
3.2 Linear Accelerations, 48
3.3 Moment and Angular Acceleration Equations, 50
3.4 Gravitational Forces, 51
3.5 Forces and Moments due to an Engine Pivoting and Throttling, 52
3.6 Aerodynamic Forces and Moments, 58
3.7 Modeling a Wind-Gust Disturbance, 70
3.8 Propellant Sloshing (Spring–Mass Analogy), 73
3.9 Structural Flexibility, 80
3.9.1 The Bending Equation, 85
3.10 Load Torques, 90
3.10.1 Load Torques at the Nozzle Gimbal, 91
3.10.2 Hinge Moments at the Control Surfaces, 93
3.11 Output Sensors, 97
3.11.1 Vehicle Attitude, Euler Angles, 97
3.11.2 Altitude and Cross-Range Velocity Variations, 98
3.11.3 Gyros or Rate Gyros, 98
3.11.4 Acceleration Sensed by an Accelerometer, 100
3.11.5 Angle of Attack and Sideslip Sensors, 101
3.12 Angle of Attack and Sideslip Estimators, 102
3.13 Linearized Equations of a Spacecraft with CMGs in LVLH Orbit, 104
3.14 Linearized Equations of an Orbiting Spacecraft with RWA and Momentum Bias, 106
3.15 Linearized Equations of Spacecraft with SGCMG, 107
4 Actuators for Engine Nozzles and Aerosurfaces Control 109
4.1 Actuator Models, 111
4.1.1 Simple Actuator Model, 112
4.1.2 Electrohydraulic Actuator, 114
4.1.3 Electromechanical Actuator, 118
4.2 Combining a Flexible Vehicle Model with Actuators, 123
4.3 Electromechanical Actuator Example, 126
5 Effector Combination Logic 137
5.1 Derivation of an Effector Combination Matrix, 138
5.1.1 Forces and Moments Generated by a Single Engine, 139
5.1.2 Moments and Forces Generated by a Single Engine Gimbaling in Pitch and Yaw, 141
5.1.3 Moments and Forces of an Engine Gimbaling in a Single Skewed Direction, 142
5.1.4 Moments and Forces Generated by a Throttling Engine or an RCS Jet, 143
5.1.5 Moment and Force Variations Generated by a Control Surface Deflection from Trim, 144
5.1.6 Vehicle Accelerations due to the Combined Effect from all Actuators, 145
5.2 Mixing-Logic Example, 147
5.3 Space Shuttle Ascent Analysis Example, 152
5.3.1 Pitch Axis Analysis, 153
5.3.2 Lateral Axes Flight Control System, 163
5.3.3 Closed-Loop Simulation Analysis, 168
6 Trimming the Vehicle Effectors 171
6.1 Classical Aircraft Trimming, 171
6.2 Trimming along a Trajectory, 172
6.2.1 Aerodynamic Moments and Forces, 176
6.2.2 Moments and Forces from an Engine Gimbaling in Pitch and Yaw, 178
6.2.3 Numerical Solution for Calculating the Effector Trim Deflections and Throttles, 180
6.2.4 Adjusting the Trim Profile along the Trajectory, 183
7 Static Performance Analysis along a Flight Trajectory 187
7.1 Transforming the Aeromoment Coefficients, 188
7.2 Control Demands Partial Matrix (CT), 188
7.2.1 Vehicle Moments and Forces Generated from a Double-Gimbaling Engine, 190
7.2.2 Vehicle Moments and Forces Generated by an Engine Gimbaling in Single Direction, 191
7.2.3 Moment and Force Variations Generated by a Throttling Engine, 191
7.2.4 Vehicle Moments and Forces Generated by Control Surfaces, 192
7.2.5 Total Vehicle Moments and Forces due to All Effectors Combined, 192
7.3 Performance Parameters, 194
7.3.1 Aerodynamic Center, 194
7.3.2 Static Margin, 195
7.3.3 Center of Pressure, 195
7.3.4 Pitch Static Stability/Time to Double Amplitude Parameter (T2), 195
7.3.5 Derivation of Time to Double Amplitude, 196
7.3.6 Directional Stability (Cn𝛽-dynamic), 197
7.3.7 Lateral Static Stability/Time to Double Amplitude Parameter (T2), 198
7.3.8 Authority of the Control Effectors, 198
7.3.9 Biased Effectors, 200
7.3.10 Control to Disturbance Moments Ratio (M𝛼/M𝛿), 201
7.3.11 Pitch Control Authority Against an Angle of Attack 𝛼max Dispersion, 201
7.3.12 Lateral Control Authority Against an Angle of Sideslip 𝛽max Disturbance, 203
7.3.13 Normal and Lateral Loads, 204
7.3.14 Bank Angle and Side Force During a Steady Sideslip, 204
7.3.15 Engine-Out or Ycg Offset Situations, 205
7.3.16 Lateral Control Departure Parameter, 206
7.3.17 Examples Showing the Effects of LCDP Sign Reversal on Stability, 209
7.3.18 Effector Capability to Provide Rotational Accelerations, 211
7.3.19 Effector Capability to Provide Translational Accelerations, 212
7.3.20 Steady Pull-Up Maneuverability, 212
7.3.21 Pitch Inertial Coupling Due to Stability Roll, 214
7.3.22 Yaw Inertial Coupling Due to Loaded Roll, 215
7.3.23 Moments at the Hinges of the Control Surfaces, 216
7.4 Notes on Spin Departure (By Aditya A. Paranjape), 217
7.4.1 Stability-Based Criteria, 217
7.4.2 Solution-Based Criteria, 220
7.5 Appendix, 224
References, 224
8 Graphical Performance Analysis 225
8.1 Contour Plots of Performance Parameters versus (Mach and Alpha), 225
8.2 Vector Diagram Analysis, 228
8.2.1 Maximum Moment and Force Vector Diagrams, 229
8.2.2 Maximum Acceleration Vector Diagrams, 233
8.2.3 Moment and Force Partials Vector Diagrams, 234
8.2.4 Vector Diagram Partials of Acceleration per Acceleration Demand, 238
8.3 Converting the Aero Uncertainties from Individual Surfaces to Vehicle Axes, 239
8.3.1 Uncertainties in the Control Partials, 241
8.3.2 Uncertainties due to Peak Control Demands, 241
8.3.3 Acceleration Uncertainties, 243
9 Flight Control Design 245
9.1 LQR State-Feedback Control, 246
9.2 H-Infinity State-Feedback Control, 248
9.3 H-Infinity Control Using Full-Order Output Feedback, 249
9.4 Control Design Examples, 251
9.5 Control Design for a Reentry Vehicle, 251
9.5.1 Early Reentry Phase, 253
9.5.2 Midphase, 261
9.5.3 Approach and Landing Phase, 268
9.6 Rocket Plane with a Throttling Engine, 275
9.6.1 Design Model, 276
9.6.2 LQR Control Design, 277
9.6.3 Simulation of the Longitudinal Control System, 278
9.6.4 Stability Analysis, 281
9.7 Shuttle Ascent Control System Redesign Using H-Infinity, 282
9.7.1 Pitch Axis H-Infinity Design, 283
9.7.2 Lateral Axes H-Infinity Design, 289
9.7.3 Sensitivity Comparison Using Simulations, 294
9.8 Creating Uncertainty Models, 298
9.8.1 The Internal Feedback Loop Structure, 299
9.8.2 Implementation of the IFL Model, 303
10 Vehicle Design Examples 305
10.1 Lifting-Body Space-Plane Reentry Design Example, 305
10.1.1 Control Modes and Trajectory Description, 307
10.1.2 Early Hypersonic Phase Using Alpha Control, 307
10.1.3 Normal Acceleration Control Mode, 317
10.1.4 Flight-Path Angle Control Mode, 329
10.1.5 Approach and Landing Phase, 341
10.1.6 Six-DOF Nonlinear Simulation, 361
10.2 Launch Vehicle with Wings, 381
10.2.1 Trajectory Analysis, 382
10.2.2 Trimming along the Trajectory, 382
10.2.3 Trimming with an Engine Thrust Failure, 385
10.2.4 Analysis of Static Performance along the Trajectory, 387
10.2.5 Controllability Analysis Using Vector Diagrams, 390
10.2.6 Creating an Ascent Dynamic Model and an Effector Mixing Logic, 393
10.2.7 Ascent Control System Design, Analysis and Simulation, 393
10.3 Space Station Design Example, 400
10.3.1 Control Design, 401
10.3.2 Simulation and Analysis, 405
Bibliography 409
Index 413
These books may interest you
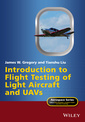
Introduction to Flight Testing 102.59 €