Description
Plasma Theory, 1st ed. 2023
An Advanced Guide for Graduate Students
Author: Rozhansky Vladimir
Language: EnglishSubjects for Plasma Theory:
363 p. · 15.5x23.5 cm · Hardback
Description
/li>Contents
/li>Biography
/li>Comment
/li>
This textbook, based on the author?s classroom-tested lecture course, helps graduate students master the advanced plasma theory needed to unlock results at the forefront of current research. It is structured around a two semester course, beginning with kinetic theory and transport processes, while the second semester is devoted to plasma dynamics, including MHD theory, equilibrium, and stability. More advanced problems such as neoclassical theory, stochastization of the magnetic field lines, and edge plasma physics are also considered, and each chapter ends with an illustrative example which demonstrates a concrete application of the theory. The distinctive feature of this book is that, unlike most other advanced plasma science texts, phenomena in both low and high temperature plasma are considered simultaneously so that theory of slightly ionized and fully ionized plasmas is presented holistically. This book will therefore be ideal as a classroom text or self-study guide for a wide cohort of graduate students working in different areas like nuclear fusion, gas discharge physics, low temperature plasma applications, astrophysics, and more. It is also a useful reference for more seasoned researchers.
1.1 Boltzmann equation
1.2 Collision operator
for Coulomb collisions
1.2.1 General expression for a flow in the velocity space caused by
collisions
1.2.2 Deceleration and diffusion of test particles cloud in the
velocity space
1.2.3 Momentum an energy loss of the test particles
1.2.4 Landau collision operator
1.3 Relativistic
collision operator
1.4 Fokker-Planck
equation
1.5 Runaway electrons
in fully ionized plasma
1.6 Distribution
function of electrons in slightly ionized plasma
1.6.1 Approximation
1.6.2 Distribution function in the electric field
1.6.3 Impact of electron-electron collisions
1.6.4 General expression for
1.7 Transport
coefficients for electrons in slightly ionized plasma
1.8 Drift kinetic
equation in a stationary electric and magnetic field
1.9 Gyrokinetic
equation
1.10 Pellet ablation in
a tokamak
2.1 Momentum equations
2.2 Transport
coefficients in fully ionized plasma. Method of Chapman and Enskog
2.3 Summary of the
results for the fully ionized plasma
2.4 Transport
coefficients in fully ionized plasma. Qualitative consideration
2.4.1 Friction caused by relative mean velocity, thermal force
2.4.2 Spitzer conductivity
2.4.3 Heat flux. Conductive and convective parts
2.4.4 Collisional heat production
2.4.5 Viscosity
2.5 Equation for
entropy
2.6 Viscosity in the
BGK approximation
2.7 Thermal force for
impurities
2.8 Finite ionization
potential effect and impurity retention in a tokamak edge
Chapter 3. Quasineutral Plasma and Sheath Structure
3.1 Quasineutrality
maintaining
3.2 Collisionless sheath
at the material surfaces
3.2.1 Electrons in a capacitor with reflecting electric field
3.2.2 Particle and energy fluxes to the material surfaces
3.2.3 Current-voltage characteristic of the sheath. Floating
potential
3.2.4 Sheath structure. Bohm criterion
3.3 Impact of electron
emission. Double sheath
3.4 Sheath in magnetic
field
3.5 Thermoelectric
current between two electrodes
Chapter 4. Diffusion in Partially Ionized Unmagnetized Plasma
4.1 Ambipolar
diffusion
4.2 Examples of
solutions of ambipolar diffusion equation
4.2.1 Decay of initial perturbation in infinite plasma
4.2.2 Positive column of glow discharge
4.2.3 Diffusive decay
4.2.4 Diffusive probe
4.3 Diffusion of slightly ionized multispecies plasma
4.4 Diffusion in the ionosphere
Chapter 5. Diffusion of Partially Ionized Magnetized Plasma
5.1 Diffusion and
mobility in magnetic field
5.2 One-dimensional
diffusion in magnetized plasma
5.2.1 Diffusion across magnetic field
5.2.2 One-dimensional diffusion at arbitrary angle with magnetic
field
5.3 Diffusion of
perturbation in unbounded plasma
5.4 Diffusion in
plasma restricted by dielectric walls
5.5 Diffusion in a
cylinder with conducting walls
5.6 Diffusive probe in
magnetic field
5.7 Experiments in
laboratory plasma
Chapter 6. Partially Ionized Plasma with Current
6.1 Plasma with net
current in the absence of magnetic field
6.1.1 Small perturbations
6.1.2 Nonlinear evolution
6.2 Magnetized plasma
with current
6.2.1 One - dimensional evolution
6.2.2 Multidimensional evolution of small perturbation in unbounded
plasma
6.2.3 Effect of conductivity recover in a weak magnetic field
6.3 Plasma clouds and layers in the ionosphere
6.3.1 Redistribution of metal ions in the polar ionosphere. Sporadic
layers
6.3.2 Active experiments with Barium clouds
Chapter 7. Transport in Strongly Ionized Plasma Across Magnetic
Field
7.1 Classical
diffusion of fully ionized plasma across magnetic field
7.2 Transport of
impurities in fully ionized plasma across magnetic field
7.3 Partially ionized
magnetized plasma with inhomogeneous neutral component
7.4 Penetration of
neutral particles into hot tokamak plasma
Chapter 8. Drift Waves and Turbulent Transport
8.1 Drift waves in
inhomogeneous plasma
8.2 Drift-dissipative
instability
8.3 Universal
instability
8.3.1 Fluid ions
8.3.2 Kinetic ions
8.4 Instabilities,
caused by the temperature gradient
8.5 Turbulent
transport caused by random electric fields
8.6 Effect of magnetic
shear on plasma instabilities
8.7 Turbulent
transport in dielectric tubes
Chapter 9. Dynamics of Fully Ionized Plasma in the Absence of
Magnetic Field
9.1 Ion acoustic waves
9.2 Nonlinear
dynamics. Self-similar solutions
9.3 Simple nonlinear
waves. Overturn
9.4 Nonlinear ion
acoustic waves with dispersion
9.5 Plasma expansion
during pellet injection
Chapter 10. Magnetohydrodynamics (MHD)
10.1 MHD equations
10.2 Magnetic field
frozen in and skin effect
10.3 MHD waves
10.4 Nonlinear MHD waves
10.5 Magnetosonic waves
with dispersion
10.6 Alfven masers
Chapter 11. Dynamics of Plasma Blobs and Jets in Magnetic Field
11.1 Plasma motion
across magnetic field in vacuum
11.2 Deceleration of
plasma jet by ambient plasma
11.3 Edge localized
modes and filaments
Chapter 12. Plasma Equilibrium
12.1. On possibility of
equilibrium in the absence of vacuum magnetic field
12.2. Equilibrium of a
pinch
12.3. Magnetic flux
surface functions
12.4. Grad-Shafranov
equation
12.5. Integral equilibrium
in a tokamak
12.6. Plasma equilibrium
in tokamak with circular cross sections
12.7. Coordinates for
arbitrary flux surfaces
12.8. Force-free
equilibrium and pinch with canonical profiles
12.9. 2D modeling of
tokamak edge
Chapter 13. Transport Phenomena in Tokamaks
13.1. Fluid regime
(Pfirsch-Schlueter regime)
13.1.1 Qualitative estimates
13.1.2. Heat conductivity
13.1.3 Plasma flows on the flux surface, density, temperature and
potential perturbations
13.1.4 Particle fluxes
13.2. Radial electric
field, poloidal and toroidal rotation
13.3. Neoclassical
transport in collisionless regimes
13.3.1 Particle trajectories
13.3.2 Ware drift
13.3.3 Estimation of transport coefficients in the plateau regime
13.3.4 Estimation of transport coefficients in the banana regime
13.4. Distribution
function in the collisionless regimes
13.4.1 Plateau regime
13.4.2 Banana regime
13.5. Particle and heat
balance equations
13.6. Transport codes
Chapter 14. Instabilities in Magnetized Plasma
14.1. Rayleigh-Taylor
instability in fluids
14.2. Flute instability
14.3. Dissipative
modifications of flute instability
14.3.1 Rayleigh-Taylor instability in partially ionized plasma
14.3.2 Flute instability in plasma contacting with metal surfaces
14.3.3 Gravitational-dissipative flute instability
14.4. Energy principle
14.5. Kink instability
14.6. Tearing instability
14.7. Geodesic acoustic
mode and zonal flows
14.8. Equatorial plasma
bubbles
Chapter 15. Magnetic Islands and Stochastic Magnetic Field
15.1. Magnetic islands
15.2. Stochastic
instability and magnetic field line diffusion
15.3. Transport in
stochastic magnetic field
15.4. Resonant magnetic
perturbations in tokamak
15.5. Simulation of
resonant magnetic perturbations effects with codes and
examples of experimental
results
Chapter 16. Improved Confinement Regime (H-mode)
16.1. drift shear and transport barriers
16.2. Transition from low
to high confinement regime (L-H transition)
16.3. L-H transition power
threshold
Builds a solid foundation in the fundamentals, explores advanced research topics, and gives illustrative examples
Covers both low and high temperature plasmas simultaneously
Designed for a two-semester course, but is also ideal for self-study
These books may interest you
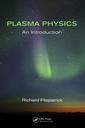
Plasma PhysicsAn Introduction 76.05 €